Sascha Roth figured that when you have cancer in your colon and lymph nodes and your oncologist calls you after business hours, two days before you're scheduled to begin a brutal five-week course of radiation therapy, it's probably not good news—especially when the first thing she says is, "Are you sitting down?"
Roth was the first patient that enrolled into a clinical trial to test a new immunotherapy drug, designed to unleash the body's natural immune response on the tumor cells, on patients with early stage cancers. The results were miraculous: One hundred percent of the patients went into total remission—perhaps the first time that's ever happened in any cancer clinical trial, according to Luis Diaz, head of the division of solid tumor oncology in Memorial Sloan Kettering's Department of Medicine and a designer of the trial. The trial suggested the new therapies, if delivered early enough, might not only obviate the need for traditional therapies notorious for their brutal side effects, like chemo, radiation and surgery. They could potentially cure the disease altogether.
Roth's doctor was jubilant: "We've reviewed all your scans, biopsies—everything," she says the doctor told her. "There is zero sign of cancer. We can't radiate you knowing there's no cancer in your body."
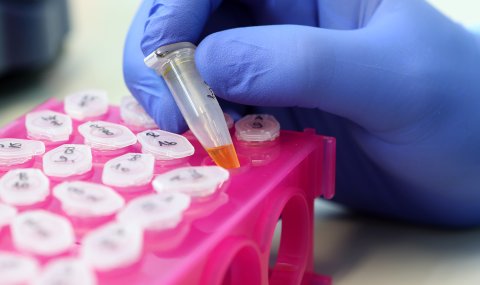
The trial, published last year in The New England Journal of Medicine, electrified the field of cancer research and held out the promise of a powerful new approach that might help relieve many people from the suffering and anxiety caused by a scourge that kills 610,000 people each year in the U.S.
So far, it's only a promise. Despite the success of Roth's and subsequent trials, immunotherapy only works for about one patient in five who receive it. Doctors, furthermore, have no way of telling any particular patient if immunotherapy will work—there's no way of foreseeing which patients will respond and which won't (though some cancers respond better than others).
In recent months, optimism has begun to yield to that old familiar feeling among cancer researchers: frustration. In the long fight against the disease, some oncologists have often felt that they are playing checkers while cancer, with its shapeshifting, mutational superpowers, is, as one prominent cancer researcher put it, playing "three-dimensional chess." In this sense, last year's trial is a tantalizing glimpse of what the future could look like—and a stark reminder of just how much further medicine has to go to make this future a reality.
Many oncologists point out that there is much cause to be optimistic. New imaging technology has recently begun to give scientists precise data on the location of proteins inside and around tumors and how they interact with one another. And advances in artificial intelligence are giving them the ability to interpret the reams of data that these new imagers are generating. Together with genetic sequencing technology, which can quickly and inexpensively determine the genetic makeup of tumors in individual patients, scientists are now gaining insights that would have been unthinkable a few years ago.
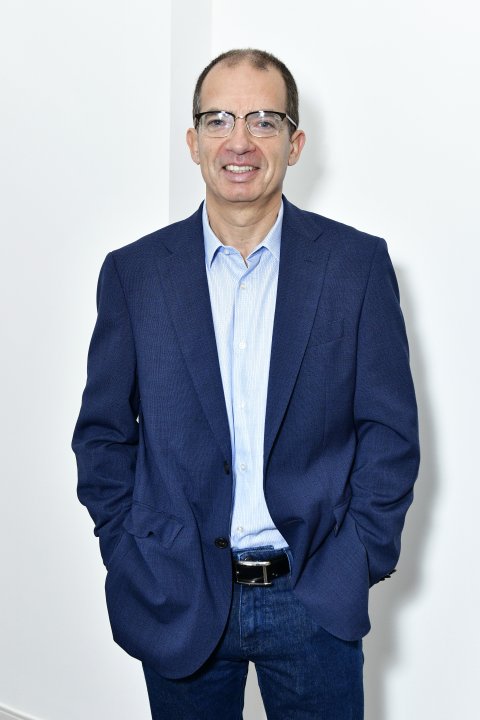
"It's now possible to take a slice of a tumor and characterize and measure every single cell, and produce staggering amounts of information," says James R. Heath, a bioengineer who heads the Institute for Systems Biology in Seattle. "These AI models can crunch these fantastically large number sets and come up with hypotheses for us. This could prove transformative."
The results may soon be improving the lives of patients. The first AI-designed anti-cancer drug is now in clinical trials, and pharmaceutical firms are using AI to develop others. Investment in AI-based drug discovery has soared: Between 2018 and 2022 it tripled to $24.6 billion. The remarkable success of the colon-cancer trials, like the one that cured Roth, could turn out to be a preview of what's to come.
Cancer's Dark Secrets
Cancer is so complex that it's beyond the capacity of the human mind to understand. Cancer cells mutate in ways that can stymie the best treatment regimes, often acting to shut down the body's immune system. To understand how they do that, and develop new methods of turning the human immune system on the attack against cancer, it will be necessary to get real data at the level of molecules about what a particular patient's cancer is doing at a particular time.
For decades, however, cancer researchers have essentially had blinders on, working with little ability to tell what was going on with the disease they were trying to treat. The fight against cancer is a story of gradual lifting of those blinders.
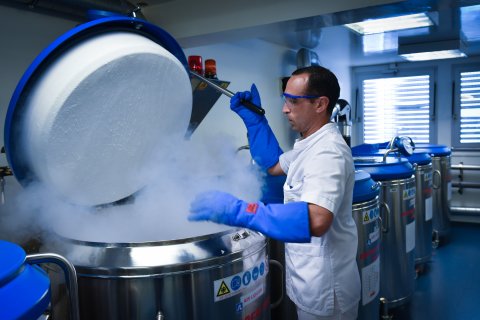
As recently as the late 20th century, many scientists didn't think the body's immune system could help against cancer. Although they appreciated the power of the billions of immune-system cells that circulate through the human body like an army on the lookout for microscopic interlopers, many had concluded that cancer cells were too similar to healthy human cells for the immune system to recognize them as invaders. That similarity is why so many cancer treatments—such as radical mastectomy, radiation and chemotherapy—rely on destroying healthy cells along with malignant ones.
By the time Luis Diaz arrived at John Hopkins Medical School in the early 1990s, however, scientists were figuring out ways of targeting cancer cells. Diaz worked in the lab of Bert Vogelstein, a pioneering cancer geneticist. Vogelstein helped demonstrate that many cancers develop as a result of mutations in specific genes, called oncogenes, that normally regulate cell growth and proliferation, along with problems in other genes, known as tumor suppressor genes, that act to destroy defective genes. He and his colleagues have since catalogued hundreds of mutations unique to different forms of cancer.
With this ability to identify genetic characteristics unique to cancer cells, scientists began developing therapies to destroy them. In 2001, the FDA approved Gleevec, the first drug to target proteins caused by a specific mutation in a specific kind of cancer—a genetic abnormality found in chronic myeloid leukemia, a rare malignant form of blood cancer. The drug, which neutralized the proteins that caused the cells to proliferate uncontrollably, raised the 10-year survival rate to 80 percent, from less than 50 percent. A whole class of new targeted genetic therapies have since followed, dramatically increasing survival rates in some cancers.
Many cancers, however, can outwit this strategy by mutating into different forms during treatment. A therapy would work for a while but its effectiveness would decline over time, to the point where the cancer would come roaring back. In most cases, scientists did not yet have data on how these mutations progressed. And even when they did, they didn't have the AI needed to interpret the data. That's what inspired oncologist Ira Mellman to re-mark that cancer plays "three-dimensional chess.""
"It is like the tumor's got this infinite range, an infinite ability to adapt to new environments," says Mellman, vice president of cancer immunology at Genentech, a San Francisco–based biotech company and maker of Herceptin and Avastin, two of the most effective targeted therapies. "We as oncologists still don't have enough tools to respond."
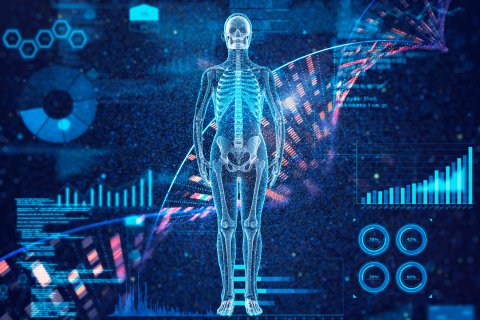
Fortuitously, however, by the time the limitations of the new targeted genetic therapies were becoming clear, a series of seminal experiments performed by pioneering immunologist James Allison revived the idea of immunotherapy and provided what many believe will eventually prove to be the ideal solution. By the early 1990s, Allison had homed in on a series of tiny proteins on their surface known as "receptors," which act like microscopic antennas, capable of recognizing foreign particles called "antigens," binding to them, and then initiating an attack. Allison had already identified a key receptor, called CD28, that functioned like "on" switches, activating the T-cell to attack. In the early 1990s, the lab of UC San Francisco immunologist Jeff Bluestone discovered that another protein called CTLA-4 switched T-cells off, acting like a molecular circuit breaker that shuts down attacks.
The CTLA-4 protein, he showed, served an important function: It protects the body from an overzealous immune system, which could cause damage to its own cells if allowed to operate unchecked. While Bluestein focused on the utility of this discovery in the fight against autoimmune diseases, Allison had a different idea: By developing a drug to neutralize CTLA-4, he wondered if he might be able to turbocharge the body's response to cancer.
To find out, Allison injected a CTLA-4 antibody into mice riddled with cancerous tumors. The anti-body was designed to seek out CTLA-4, glom onto it and jam the switch, so it couldn't turn off T-cells. The technique worked better than Allison had imagined. We now know why: Many cancers develop mutations that use CTLA-4 to switch off the immune response.
Allison's experiment was among the first to demonstrate it was possible to counter the mutational moves of cancer, intervening in the molecular chess match between the body's own defenses and cancerous tumors. In 2000, doctors administered Allison's antibodies to 14 patients stricken with terminal metastatic melanoma, most of whom were under hospice care. Three of the patients went into complete remission. The first immunotherapy treatment for melanoma was approved in 2011.
The treatment ushered in a new paradigm in can-er treatment. In the years that followed, oncologists would discover several other cellular circuit break-rs that could be jammed with drugs. They could be used in different forms of cancer—including bladder, breast, head, neck Hodgkin's lymphoma, kidney, melanoma and non-small-cell lung cancer—usually in conjunction with chemotherapy.
But nagging questions remained. Why did immunotherapy only work in two of the 14 patients in that initial trial? The question today: Why does immunotherapy, in general, work in some kinds of cancers, and only some of the time?
A Million Other Tricks
In the 2010s, Diaz and his colleagues at Johns Hopkins speculated that there could be many factors influencing the response of specific cancers to drug therapies. They were beginning to realize that the progress of cancer is influenced by myriad factors, which, many researchers believe, will ultimately require AI to figure out.
One factor in particular stood out to Diaz and his colleagues. The drugs seemed to work best in cancers characterized by an abnormally high number of mutations, such as melanoma and lung cancer. (In melanoma, mutations are caused by exposure to sunlight; in lung cancer, by exposure to cigarette smoke).
The idea made sense, since mutations would be likely to draw the attention of the immune system, which is designed to hunt out foreign-looking pathogens that don't belong in the human body. In these cancers, they theorized, T-cells were primed to attack—but cancer had immobilized them before they could do so, by flipping Allison's CTLA-4 circuit breaker. The drugs turned the T-cells back on.
Diaz hit upon a way to test this theory during a chance encounter in an elevator. His colleague, Drew Pardoll, was running the first large clinical trial testing the effectiveness of the new immunotherapy drugs on patients with colon cancer. Pardoll had told Diaz that none of the patients enrolled in the study had responded to the therapy. Later, in the elevator, he casually mentioned that he'd been mistaken: A single patient had responded.
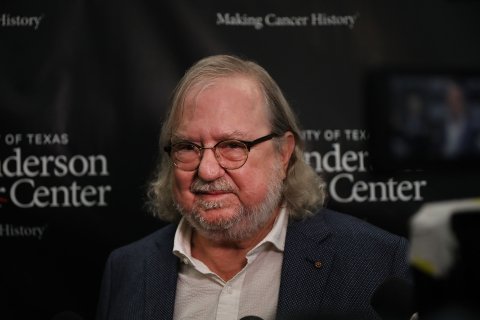
For Diaz, a lightbulb went off. He asked Pardoll if, by any chance, the patient happened to have a rare condition that, in five percent of colon cancer patients, causes their tumors to develop an unusually large number of genetic mutations?
The condition, known as Lynch Syndrome, arose from a deficiency in the cellular machinery that corrects mutations in cells that accumulate as they divide. While normal colorectal tumors have roughly 70 mutations, those with Lynch Syndrome have, on average, 1,700 mutations. Pardoll later confirmed that Diaz's hunch was correct: The one patient who responded to the therapy had Lynch Syndrome. "It lit a fire under us to test the hypothesis," he says.
In 2013, Diaz launched the first of several clin-ical trials, seeking out patients in the final stages of metastatic cancer. He enrolled 32 patients with colorectal cancer, and nine with other types of sol-id tumors. The results were remarkable: Forty per-cent of the colon-cancer patients and 71 percent of those with other types of cancer responded to the drugs. None of those who did not have the problems in correcting DNA mutations responded.
Diaz expanded the study to include 86 patients with 12 different cancer types, all of whom had "mis-match repair-deficient cancers," the condition caused by Lynch Syndrome in which the body has lost the ability to correct a specific type of error in DNA replication (Lynch Syndrome refers to the heritable form of the condition, but it can also develop due to mutations that occur after birth). This time, rather than recruiting patients with metastatic cancer who were headed to hospice, he focused on recruiting patients newly diagnosed with metastatic tumors. The outcome: Three-quarters of the patients responded and 18 went into full remission.
The results, published in 2017 in the journal Science, along with similar results in four other studies, led the FDA to approve the drug as a treatment for all types of late-stage cancers with the types of genetic mutations caused by those with "mismatch repair deficient cancers," regardless of where in the body the cancer originated. It was the first "tumor agnostic" approval in the agency's history. Since then, the FDA has approved three other tumor-agnostic cancer therapies—larotrectinib, entrectinib, and dostarlimab.
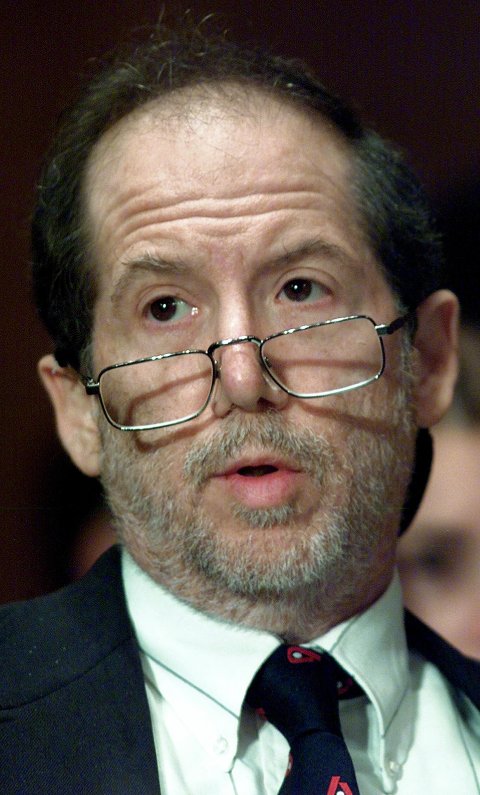
Diaz was struck by how much better the results had been simply by treating patients a little earlier. So in 2017, when he moved to Memorial Sloan Kettering, he began discussing the idea of trying the approach out on patients in early-stage cancer with Andrea Cercek, a rectal cancer expert. It would be, in some ways, a bold decision. Traditionally, experimental immunotherapies had been reserved for the one-third of patients with metastatic disease, in which the cancer has begun to spread from the original site of the tumor. The other two thirds received the traditional tools of radiation, chemotherapy and surgery. But the downside of these treatments was so great for colorectal cancer patients—infertility, impaired sexual function and removal of the rectum—that Cercek and Diaz agreed the new approach was worth a shot.
The results exceeded even his wildest expectations. "Every single one avoided chemotherapy, radiation and surgery. And probably the most exciting part now is that three of those patients have had babies," he says. But as is often the case with cancer, even success raises many new questions. For one, why was this therapy so successful?
"The tumor's not coming back," says Diaz. "From a scientific standpoint, that's unbelievably interesting. And that uncovers a lot of questions, like, what the hell's going on here?"
Treating the cancer early is clearly a factor, but that doesn't entirely explain it, says Diaz. He isn't sure what made the difference, but he has some ideas.
Younger tumors have less time to develop mutations and to burrow into surrounding tissues, changing them in ways that might make it harder for immune cells to reach them. Also, some tissues, such as bones, liver and brain, are known to have protective immune "traffic cops," called myeloid suppressor cells, designed to keep immune responses in check. Is it possible that there is something about the location of the cancer, in the rectum, that is permissive for a very strong immune response?
Bad Neighborhoods
One of the most promising areas of investigation in oncology is the idea that the tumor "microenvironment"—the ecosystem of proteins and other molecules surrounding individual tumors—may be a key factor in determining the effectiveness of immune therapies. Taking the tumor microenvironment into account vastly adds to the complexity of the problem. It's not only the billions of proteins that are important but also all the ways they interact with one another. And it puts a premium on new imaging technologies that can see into this microenvironment.
Padmanee Sharma, an immunologist and oncologist at the MD Anderson Cancer Center in Houston, Texas, has experienced the power of the new technologies first hand. In 2012, Sharma, a long-time collaborator of Allison's (the two are also married), began cataloguing thousands of proteins present in tissue samples taken from the tumors of patients enrolled in clinical trials. The idea was to find new molecules associated with "hot" tumors—those in which T-cells penetrate and attack the cancer—and "cold" tumors, those where immune cells fail to gain traction. Almost immediately they identified a molecule, called inducible T-cell costimulator, that makes T-cells far more likely to attack tumors. But identifying the presence of specific molecules can only take you so far.
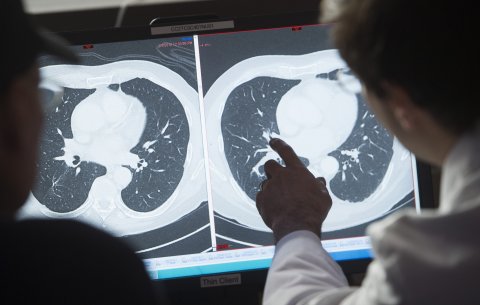
In 2018, Garry Nolan, a Stanford University immunologist, invented an imaging technology to figure out precisely where these proteins are located in a tumor—in other words, to map the cancer cells' microenvironment. Called CODEX (for co-detection by indexing), the technology allows researchers for the first time to track where these individual proteins are arrayed in space and how they are interacting with one another.
Nolan used light-sensitive "tags" attached to antibodies designed to seek out and attach to specific proteins (such as CTLA-4, ICOS or any of the other proteins present on the surface of immune cells or released by cancer cells). Each one of these antibody tags will fluoresce in response to specific wavelengths of light. Overlaid across the tumor sample are a series of DNA barcodes that break the sample into a giant grid pattern, like a tic-tac-toe board.
Each one of these tic-tac-toe squares is exposed to a sequence of different wavelengths of light. Imaging technologies record which proteins fluoresced in response to each wavelength, and where they are located in the barcoded grid pattern. These responses are then layered on top of one another and used to construct an accurate map of which proteins are present and where they are arrayed in relation to one another.
Data on how proteins are positioned in a tumor and how they interact with one another is already revealing some surprising insights. Many immune responses are proceeded by a specific and well-observed phenomenon in the body's lymph nodes: T cells come together with a different type of immune cell called a B cell. The two key cells are "exchanging information about the presence of foreign invaders within the body," so they know what targets to attack, says Sharma.
kUsing CODEX, Sharma has spotted this same microscopic phenomenon—usually only seen in the lymph nodes—occurring in the tumor micro-environments associated with an effective immune response. Just outside the tumor, T-cell and B-cells would cluster together and form what oncologists call "tertiary lymphoid structures," a mobile headquarters of sorts that seems to be where the immune system mounts a strong, successful response.
"This is not something that we had envisioned before," she said. "But these immunotherapy agents can create these tertiary lymphoid structures. And then that seems to correlate with patients responding to therapy. Which raises a lot of interesting questions. What are the signals that drive the tertiary lymphoid structures? Can you imagine if I understood what those signals were? Could I not just make every patient's tumor form those structures?"
In other words, if Sharma could figure out the microscopic signals that cause the T-cells and the B-cells to converge just outside the tumor and form these mobile headquarters, she could vastly increase the likelihood the immune system will launch a successful attack.
At Stanford, Nolan has also used CODEX to gain new insights into how some tumors in head and neck cancers prevent T-cells from attacking them. On images, he has spotted thick barriers consisting of the kinds of structural proteins that form the connective tissues and scaffolding of the cells that make up our bodies. These macromolecules, which comprise what's known as extracellular matrix, may prevent immune cells from infiltrating the tumors. This insight suggests a new way to boost immune response by introducing enzymes designed to degrade the barriers. It's an interesting theory that Nolan wants to test.
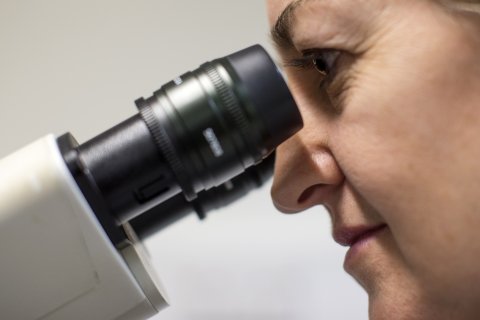
These observations are just the beginning of what scientists will be able to learn from the staggering amount of data—literally, millions of variables—that CODEX can produce. In theory, CODEX makes it possible to reverse engineer, down to the molecule, everything that happens inside a tumor.
Digesting reams of CODEX data is where artificial intelligence comes in. AI is quickly becoming a central tool in cancer research. AI could identify which cells, or combination of cells, are associated with patients who respond to treatments and patients who do not respond to them. It could then suggest solutions—and even design drugs that eliminate whatever it is that is preventing a patient's immune system from defeating the cancer.
"You would look for patients who don't respond, and then you'd go in and you'd say, okay, well, what cells are around the T cells?" says Heath from the Institute for Systems Biology.
Already some companies are using AI to comb through relevant data on cancer. At Insilico Medicine, founded in 2014, computer engineers have trained its AI on publicly available data, including on biopsy samples from hundreds of thousands of patients. The company can now rank 20,000 genes based on their specific contribution to cancer and model biological pathways to see which ones are driving the cancer, what may be causing the disease and where drugs might best work to fight it. Last February, the company identified, synthesized and tested 71 small molecules, each one with a unique structure designed to block a key don't-eat-me signal used by tumors to evade immune detection. The most promising candidates recently entered early-stage clinical trials.
The company is now working on miniaturizing its 1600-square-foot, six-room fully automated AI-run robotics lab, called Lifestar One, into a two-room mobile unit that can be placed on-site at hospitals to provide personalized therapies for individual patients based on their tumor profiles, and validate each therapy by testing it on human tissue cultures or in lab mice, with robots doing all the mixing and lab work. The project is one possible way of quickly responding to cancer with effective counter-measures—giving doctors a fighting chance to beat cancer at three-dimensional chess.
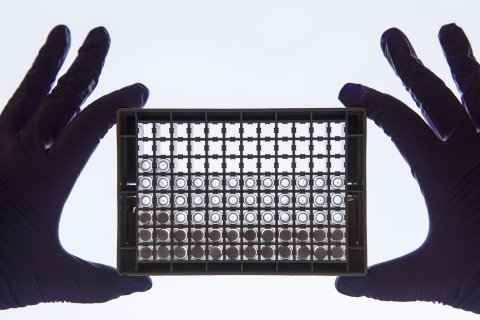
The Chase
Cancer medicine has had so many disappointing moments in the last few decades that it may seem like a Quixotic pursuit. But the field has made substantial progress. Whereas the overall age-adjusted cancer death rate in the U.S. rose steadily higher throughout much of the 20th century, it peaked in 1991 at 215 per 100,000. As of 2020, the death rate had dropped 33 percent to about 144 per 100,00—almost four million fewer deaths over the course of that 29-year period. Reductions in smoking account for some of those improvements, but not all. Another factor has been advances in treatment and early detection—largely in lung, colorectal, breast and prostate cancers, the most common types.
Diaz and Sharma believe that the best shot at getting the death rate lower lies in early detection—when cancer has had less of a chance to accumulate mutations, wall itself off in an inaccessible microenvironment and disguise itself using techniques that scientists are just beginning to understand.
The MSK colorectal cancer trial is just one of several in recent months that have demonstrated that new immunotherapy drugs seem to work far better in some early-stage cancers than anyone expected—so well they obviated the need for surgery and radiation, allowed the preservation of organs and caused complete remission. In recent years, scientists, like Dr. Vogelstein and colleagues, have come up with increasingly sensitive diagnostic tests that can detect microscopic DNA fragments associated with cancer in the bloodstream months and even years before they would otherwise become apparent.
The FDA has approved liquid biopsies, which can detect small pieces of DNA from dead or broken-down tumor cells. The tests are now most commonly used to monitor the success of some cancer treatments and monitor disease progression in patients with lung, breast, prostrate, colorectal ovarian and other solid cancers. Since the amount of tumor DNA in the circulatory system drops as the tumors shrink, they are not yet sensitive, reliable or specific enough to be used to screen or diagnose cancers. But they have shown promise as diagnostic tools in some early-stage trials now underway. In some cases, AI plays a role in interpreting the diagnostic data. Many oncologists believe, and hope, that early detection will eventually transform the treatment outlook for many cancers in the years ahead.
When—and if—immunotherapy approaches will provide an effective treatment option for most cancer patients is unknown. Diaz is skeptical. He believes that some cancers will likely never respond to immunotherapy. But he is no cynic. He and his team recently used genetic testing to identify patients with Lynch Syndrome before they had developed cancer. When they applied the therapeutic immuno-therapy protocol to these patients, they demonstrated they could prevent cancer from occurring altogether. The work was published in October in Nature Medicine.
Meanwhile, Diaz celebrates each patient in his trials who is fortunate enough to respond to immunotherapy treatments. "It is a feeling that you want to hap-pen over and over again," he says. "It's unbelievable."
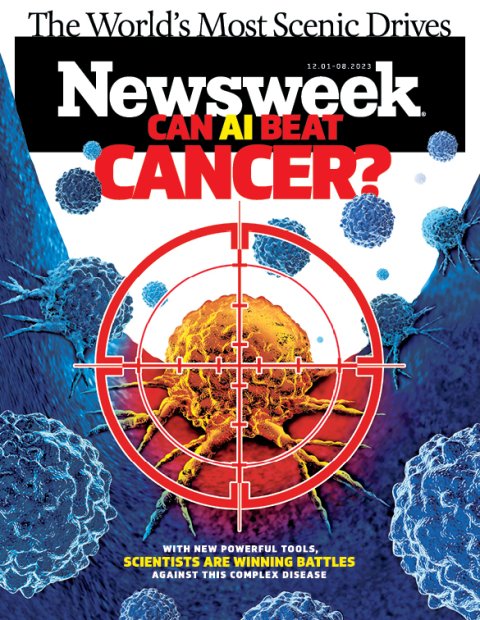